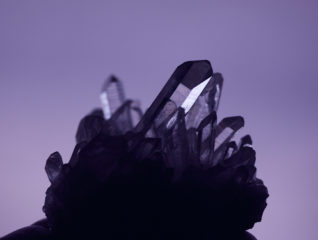
What Is Shungite?
- Book Sample
Introduction to Shungite
This article was adapted from the first chapter of Healing with Shungite by Jessica Mahler.
Definition of Shungite
Shungite is classified as an amorphous (meaning it cooled extremely fast) metamorphic mineral. It started off as a different type of rock altogether, but extreme heat and pressure within the earth’s crust transformed its mineral makeup to become what we know as shungite today. Geologists believe that shungite first formed during the Paleoproterozoic Era, the longest era in the earth’s geological formation, 2.5 to 1.6 billion years ago. It was first discovered in seventeenth century Russia in Karelia, near Shun’ga village, where shungite’s name is derived from. Shungite is only found naturally in northwest Russia, in the upper Zaonezhskaya Formation, just northeast of St. Petersburg.
The Three Different Types of Shungite
There are three types of shungite.
TYPE I (ELITE SHUNGITE)
- Referred to as “silver shungite”
- Black in color, with a silvery shine
- Made up of 98 percent organic carbon, with trace amounts of nitrogen, sulfur, oxygen, and hydrogen
- Manually excavated
- Easily identified by its conchoidal fractures, smooth curves resembling a round snail shell, a physical attribute from cooling too quickly when it formed
- Sold in its natural shape
- Lightweight
- Extremely fragile
- Only available as a raw stone but sometimes sold as jewelry in its raw state
- The rarest shungite, making up only 1 percent of all shungite found in the world
- Due to its high carbon makeup, the most powerful and effective shungite
- Best used for water purification
TYPE II (PETROVSKY SHUNGITE)
- Grayish-black in color
- Made up of 64 percent organic carbon, 3.5 percent nitrogen, 3.5 percent oxygen, 6.7 percent hydrogen, and up to 3.3 percent ash
- Industrially mined
- Much sturdier than elite shungite
- Heavy in weight
- Easily shaped and polished, so it’s used for jewelry and figurines
- Considered a transitional stone because it has all the healing properties of elite shungite and is almost as effective
TYPE III (REGULAR SHUNGITE)
- Black in color
- Made up of 30 to 50 percent organic carbon; up to 56 percent silicon dioxide; 4.2 percent water; 4 percent aluminum oxide; 2.5 percent iron oxide; 1.5 percent potassium oxide; 1.2 percent magnesium oxide; 1.2 percent sulfur; and trace amounts of calcium oxide, sodium oxide, or titanium oxide
- Sturdier than both Petrovsky and elite shungite
- In terms of weight, the heaviest type of shungite
- Easily shaped and polished, so commonly used for jewelry and figurines
- Commonly referred to as “shungite rock” since this type contains a much lower percentage of organic carbon than the other two
The Elemental Makeup of Shungite
It’s shungite’s high carbon makeup that gives the mineral certain catalytic abilities, making it especially good for electroconductivity and chemical resistance. This is the magic behind shungite’s EMF-fighting capabilities. We’ll discuss more about EMFs in Chapter Four.
But let’s not get too far ahead of ourselves. To understand how shungite came to be, we have to start at the beginning.
All crystals start out as magma, that molten fluid found bubbling beneath the earth’s crust. When magma makes its way above ground, it’s considered lava, but the atmosphere is no longer hot enough to keep it in its liquid form. The cooling process of lava is what creates minerals or igneous rocks. All minerals start out as igneous rocks, but not all igneous rocks are the same. This is because magma contains many different mineral-forming elements.
Magma is the substance that created the surface of the earth. The movement or motion of magma has no ordered state. From a scientific point of view, it’s believed that 4.5 billion years ago, planet Earth started out as a gigantic cloud of cosmic dust and gas. The force of gravity drew these particles together, eventually forming a blazingly hot liquid sphere. Over time, the temperature of the sphere decreased and its composition began to change. It’s believed that around this time—about 3.5 billion years ago—the earth’s magnetic field began to form. Eventually light matter and heavy matter separated—like oil and water—creating the different layers of the earth (core, mantle, crust), and the surface began to cool, creating a thin outer shell. This shell had many cracks in it; magma and steam forced their way through these cracks to create larger, thicker layers of rock that make up the earth’s crust. With mantle flowing beneath the crust, large plates of rock (tectonic plates) began to shift: friction and collisions gave rise to mountains, volcanoes, and earthquakes, which shaped the surface of the earth.
From chaos, order was created.
Different substances commingle in magma, which informs us that the hot liquid is not a homogeneous substance; its makeup varies in different areas of the earth, which helps us understand why many different types of minerals are formed by magma. It’s also the reason that distinctive minerals are only found in certain parts of the world.
Other factors also help determine the fate or distinction of a mineral. Once the mineral makes it to the earth’s surface, outside forces like wind and water cause it to erode. Water in particular can completely dissolve certain mineral-forming elements, which then transforms the mineral’s composition. If an igneous rock is weathered by the elements in a way that has changed its mineral makeup and/or if it’s transported away from where it was created and deposited elsewhere via the wind or a body of water, then it’s considered sedimentary rock.
And yet another type of rock is metamorphic—rock that has been transformed by extreme heat and pressure without becoming molten. The metamorphic process reorganizes the original structure of igneous or sedimentary rocks to create a stronger, more stable rock. This type of rock formation tends to occur deep within the earth’s crust or at the site of colliding tectonic plates. When existing igneous and sedimentary rocks get folded back into the earth’s crust, the increase in pressure causes them to restructure into new chemical compounds. Some substances from a rock’s original makeup may quite literally get squeezed out; these compressed minerals accumulate to create new, more resistant minerals. You can actually see how a rock’s composition has bent under pressure in the striations of color in metamorphic rock like tiger’s-eye, lapis lazuli, and marble.
Time is also a factor. The longer the solidification process takes, the larger the crystal can grow; if the process is fast, the crystals are small. Sometimes the cooling happens at such a rapid pace that there is no time for crystalline structures to form, and the byproduct looks more like rock than the “ice” the Greeks referred to. It can take eons for a crystal to form, or mere seconds. Some are solidified via heat, others through freezing temperatures.
At its most basic, a crystal is a naturally occurring solid. Each type of crystal has its own precise atomic arrangement in a geometric crystal lattice that makes it unique to other classifications of crystals. Its organized structure is what provides its stability and the regularity of flow of any electromagnetic energies that pass through it. This internal structure defines a crystal.
While many different types of crystals may be formed out of the same mineral or combination of minerals, each type crystallizes in its own unique way. The same type of crystal may have several different colors or external forms so that, from the outside, it may seem as if you are looking at different minerals. But in the crystal kingdom, it’s the internal structure or lattice that determines how we classify it.
The atoms and molecules that make up crystals are tightly packed; the pressure of the electromagnetic force of the earth leaves no space to be wasted in their makeup. The most efficient way to pack atoms into solid matter is an organized system of repeating shapes. The repeating shape is known as a unit cell. The internal structures of cubic crystal systems are made up of repeating unit cells stacked on top of and adjacent to each other.
Seven geometric shapes are used for crystal classification: square, rectangle, hexagon, triangle, rhombus, parallelogram, and trapezium. If you were to throw a crystal with force at the ground, you would see that it breaks into a similar shape with similar edges. So smoky quartz and calcite, both trigonal in makeup, would break in triangular shapes; sodalite, with its cubic arrangement, would shatter into square pieces. Crystals with a lattice break into smaller, similar shapes because of their strong, interlocking, repeating internal geometric structure.
Amorphous minerals, however, are an exception to the rule. Where the geometric crystalline structures have order, amorphous minerals lack an organized internal structure. This occurs when a crystal’s formation process is too fast, lacking time for the atoms to organize themselves in an ordered fashion as it rapidly coagulates into solid matter. It could also be that there are too many different substances that come together to form the rock.
Since shungite is classified as an amorphous metamorphic mineral, by definition it would lack any kind of organized internal structure. But one of the reasons why shungite is so special is that it’s been found to be made up of fullerenes, also known as C60, or carbon 60. Fullerenes are composed of twelve pentagonal and twenty hexagonal faces, which exist together in their molecular makeup to form a geodesicdome-like structure reminiscent of a soccer ball.
Named after the futurist and inventor Buckminster Fuller, who patented the geodesic domes made famous by the 1967 World’s Fair in Montreal and Disney World’s Epcot Center, this type of molecule wasn’t discovered until 1985 by scientists Robert Curl, Harold Kroto, and Richard Smalley, who would go on to win the Nobel Prize in Chemistry in 1996 for their discovery.
Inspired by Kroto’s theory that long carbon chains existed in the atmospheres of stars, this team of scientists conducted experiments designed to simulate the gas flow emanating from aging, carbon-rich stars. In doing so, they created an artificial, synthesized structure in their laboratory that seemed alien to earth’s atmosphere. Because the extremely high temperatures and carefully controlled gas pressures needed to create fullerenes were so precise, it was believed that only a laboratory could house these perfect fullerene-making conditions. In 1992, though, natural fullerenes were accidentally discovered in shungite by geochemists at Arizona State University at Tempe2 using a high-resolution transmission electron microscope, an instrument capable of recording images of individual molecules. In essence, humans had “created” a molecule that already existed; it just hadn’t been discovered in nature yet.
Why is this important? Because understanding the structure of a crystal also helps us to understand why and how it works; in shungite, it’s the fullerenes in particular that allow it to be so effective in absorbing EMFs as well as anxiety, which we’ll look at in more depth in Chapters Four and Seven.
The Formation of Shungite
To understand the origins of shungite, we have to go back to our planet’s formation story. It’s believed that water-rich meteorites hit Earth’s surface as the crust was carving itself out, which introduced the first water into Earth’s atmosphere. Water condensation allowed the earliest elements of life to be introduced to the planet.
These first life forms, which evolved 3.8 billion years ago, were prokaryotes, cells that lack a nucleus or organized internal structure. They did, however, contain what’s since been considered the origin of life: a single strand of DNA. Feeding on carbon compounds that accumulated in the early oceans, bacteria ultimately formed. These organisms developed to use the sun’s energy and sulfides to generate their own energy, creating cyanobacteria, what we know today as blue-green algae, Earth’s first oxygen producers by way of photosynthesis. Over time, enough oxygen accumulated in Earth’s atmosphere to allow for the evolution of oxygen-metabolizing organisms, helping to jump-start the evolution of new, more complicated life.
Evolution didn’t happen overnight, though. It took roughly 1–1.5 billion years for prokaryotes to develop into eukaryotes, cells with a defined nucleus and organelles. Talk about a glacial pace.
Shungite formed about 2.2 billion years ago. It consists of decomposed prokaryotes mixed with mud and silt to form kerogen, now the most abundant form of organic matter on Earth. These sediments slowly sank into the soil and, due to Earth’s pressure, were compressed and transformed into rock. Geothermal heat and subterranean volcanic activity caused the organic materials to liquefy, transforming them further into simpler substances: hydrocarbons in the form of petroleum or bituminous shale and coal. This fluid spread out over 3,475 miles of land in what’s considered today to be the Russian part of Karelia (the Karelia region straddles the border of Finland and northwest Russia), eventually forming shungite through the petrification process. Scientists believe that the petrification process allowed the natural molecular structure of fullerenes to form.
Because of their unique molecular structure, fullerenes, one of several classifications of carbon allotropes, are used as antiviral agents. An allotrope is an element, like the carbon in fullerenes, that can configure itself in such a way that its structure and properties change, even though its composition remains the same. Fullerenes’ unique cage-like structures allow them to both carry needed molecules and trap dangerous substances in the body, and then to remove them. This makes shungite very effective in reducing the amount of electromagnetic radiation in and around a certain space.
In fact, research has shown that fullerenes are particularly potent in combating the flu, inhibiting HIV-1 replication, and suppressing herpes and other viral infections resistant to existing drugs.
Why are these cage-like structures so efficient at trapping dangerous substances? Because of their high carbon makeup. Considered the magic of life, carbon is necessary for all life-forms. All living organisms contain carbon; it exists in nearly every compound in our bodies and every function our bodies carry out. We need it to live, grow, and reproduce. Carbon is especially unique in its ability to bond to other carbon molecules, as well as to form up to four covalent bonds between atoms or molecules. A covalent bond occurs when two atoms or molecules share an electron, enabling the atoms or molecules to stick together, which also stabilizes and strengthens them.
Electron-deficient in nature, fullerenes react easily with free radicals—unstable atoms that can damage cells, proteins, and DNA. Acting as antioxidants—molecules that neutralize free radicals—fullerenes’ cage-like structure attracts and traps the free radicals and then transforms them into a neutral compound. This ability has made fullerenes popular in cosmetics technology as an anti-aging and anti-damage agent.
Healing with Shungite
Harness the restorative power of the shungite stone with this easy-to-read manual for cleansing energy at work or home, guarding yourself from EMFs, soothing anxiety and stress, and many more practical therapeutic solutions for healing with this incredible crystal. Many crystals and protective stones offer a variety of health benefits, but
Learn more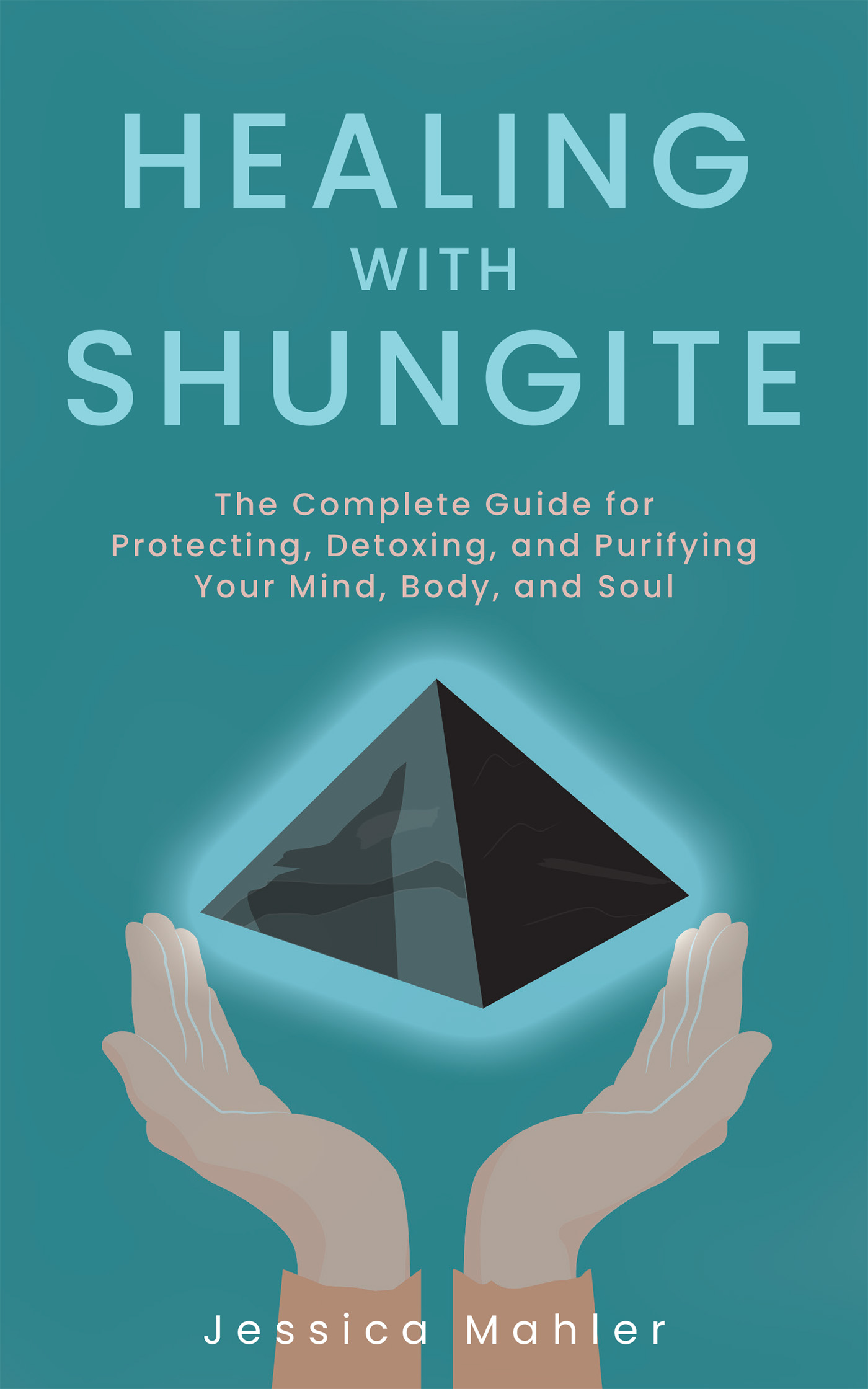